Disclaimer: Nothing that follows is investment advice. Please see our “Terms” page link below for more information regarding this website.
Our readers know that since the launch of Segra Resource Partners in 2018, Segra Capital has been wholly dedicated to studying and investing in the nuclear industry and nuclear fuel cycle. What some of you may not know is that we have spent the last several months preparing to launch a sector dedicated venture capital fund to take advantage of what we see as a generational investment opportunity in nuclear-related private markets. We view the venture capital opportunity as an extension of our hedge fund, where we can leverage our years of industry knowledge and contact network to drive a highly differentiated deal pipeline and diligence process for limited partners. Our new fund will target companies driving innovation across the nuclear sector and will have a relatively open mandate to invest in Fission (advanced light water reactor, “LWR” and non-LWR technology), Fusion[i], fuel cycle companies, commercial applications and the service and support opportunities needed to help next generation nuclear designs succeed and scale.
Today, a dual focus on decarbonization and energy security is driving a recommitment to nuclear energy across the globe. This trend, which began several years ago, has accelerated dramatically as a result of recent geopolitical events. What we are most excited about is that this top-down macro demand story is being met with incredibly exciting micro developments as companies move to commercialize next generation nuclear designs this decade. Despite this compelling set up, we continue to see the nuclear industry as underfollowed by large institutional investors who lack the expertise and experience to deploy capital effectively in what remains a highly technical and insulated industry – for Segra, this is a fantastic opportunity.
Advanced Nuclear Overview
So, what is “Advanced Nuclear” and why are we so excited about it? The term Advanced Nuclear is generally associated with a new generation of nuclear reactor designs being commercialized today. While some consider only non-LWR technologies as advanced reactors, we see significant opportunities for advanced LWR Small Modular Reactors (“SMRs”) as well as other SMR and microreactor technologies. Advanced nuclear designs build on the excellent track record of our current nuclear industry along with decades of research and development by some of the nation’s brightest minds through our National Labs programs. In addition to being smaller and more operationally flexible, many reactor designs utilize different fuels, coolants, moderators, and processes to provide solutions current nuclear plants cannot[ii].
Each design varies, but broadly speaking some of the potential advantages of advanced technologies are:
- Greater scalability with improved economics through modular build plans and factory fabrication,
- Lower initial capital investment and significantly more competition among part manufacturers and suppliers (an additional cost mitigator),
- Larger addressable markets driven by siting flexibility and heat applications,
- Load following capability to help balance intermittent renewables on grid,
- Inherent safety by design utilizing passive systems and lower operating pressures, and
- For many designs, significantly reduced nuclear waste profiles with some designs able to use existing nuclear waste (or our preferred term – “spent nuclear fuel”) as fuel!
The advanced nuclear technologies being commercialized today will not only provide reliable, baseload, carbon-free power to the electrical grid – they also have the ability to solve many of the energy transition’s hard decarbonization problems, such as industrial heating (which the graph below shows is 90% generated by fossil fuels today) and the production of clean synthetic fuels. These essential, but hard to decarbonize, parts of the global economy are unlikely to be solved in a renewables-only climate plan (see a recent OECD-NEA study on this topic here).
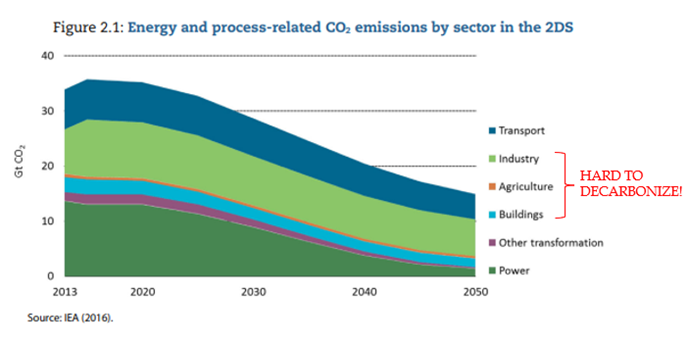
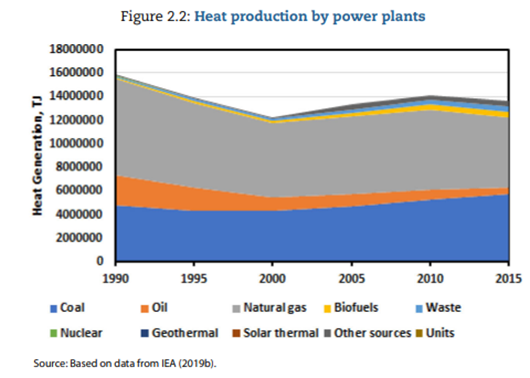
What’s more – the new technologies we are focused on are not science projects. Many of these technologies have been operated as commercial or test reactors for decades in the US and abroad. Despite many of these designs being technologically de-risked, we are just now seeing the combination of top-down government climate policy paired with customer demand (from both utility and corporate end users – recent examples include Dow Inc., Canadian Oilsands Companies, Steelmaker Nucor and a series of Korean Industrial Conglomerates) which will allow them to commercialize and scale. By our estimates we should see a dozen different advanced reactor designs built in North America and Europe during the next decade, with many more early-stage opportunities in the development pipeline.
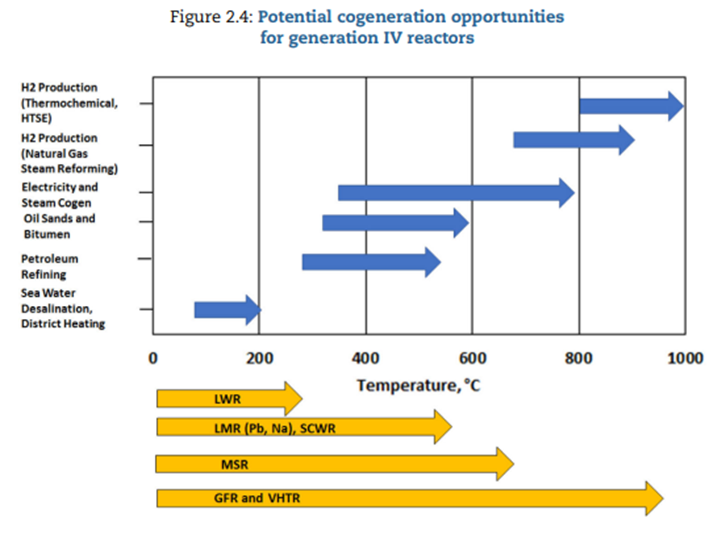
While a deeper dive into the advanced nuclear industry is beyond the scope of this post, we would direct interested readers to the Nuclear Innovation Alliance’s recent Primer and Compendium which we were happy to review prior to publication (Art took part in the media event around the release which can be seen here). They are a great resource for investors approaching advanced nuclear for the first time.
How big is the opportunity?
Projections for advanced nuclear deployment vary by study but, given the unique solutions that many of these reactors provide, the addressable market is simply massive. We have reviewed dozens of energy market studies over the years and have two major takeaways: (1) almost every credible study targeting net-zero and optimizing for system level cost of decarbonization include a significant increase in nuclear power, and (2) the more complex and developed a study’s parameters on generation deployment (examples include: incorporating considerations around zoning for wind, solar and large scale transmission lines or factoring in projected efficiency / capacity factors for renewables by deployment site rather than using a global assumption), the more models tend to favor new nuclear over competing options. There are clearly many modeling factors at play, such as assumed learning rates for new technologies, resource prices and materials limitations, etc. but, as a recent ClearPath study on wind deployment in Iowa pointed out, oversimplified modeling of deployment white space can lead to policies and projections which don’t pass a basic smell test.
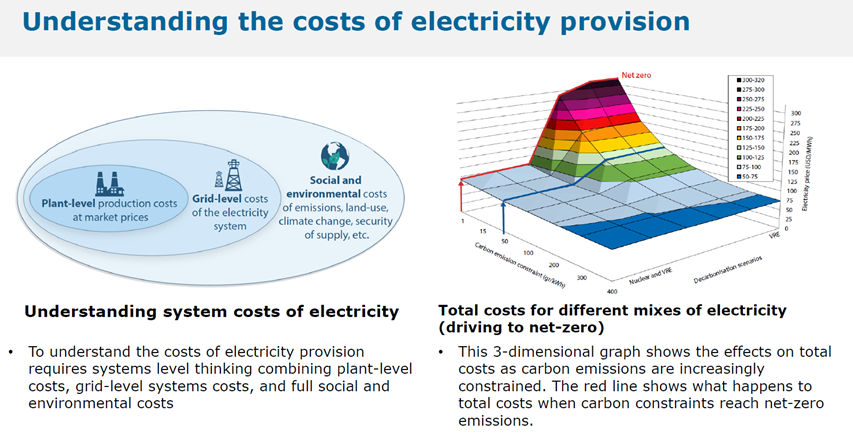
We would point readers to recent studies done by the Breakthrough Institute (peer reviewed by our Chief Technical Analyst, Brett Rampal) and Vibrant Clean Energy (links to the studies here and here) which outline the significant contribution Advanced Nuclear can make to the energy transition. The factors which drive adoption / build rates are relatively straightforward, but include access to capital, assumed learning rates as designs transition from first-of-a-kind to Nth-of-a-kind, and modelling assumptions around alternatives such as wind, solar and storage deployment. While we’ll admit that forecasting generation technologies with certainty out to 2050 is fraught with challenges, it is clear to us that nuclear energy is very much a growth industry with significant right-tail risks to current consensus forecasts.
U.S. Aggregate Generation Mix – Nominal Unconstrained Scenario
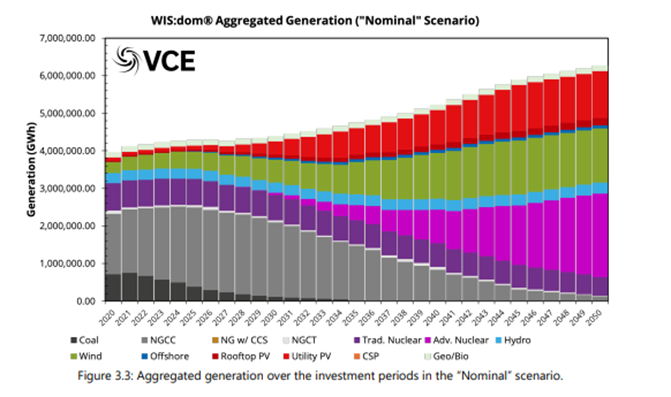
Advanced Nuclear’s Impact on the Nuclear Fuel Cycle
We would be remiss not to highlight how our work on the advanced nuclear opportunity set has impacted our modelling of the nuclear fuel cycle. While new reactor technologies employ a variety of different fuel designs (and some do incorporate different natural resources such as Thorium), the vast majority continue to depend on the uranium fuel cycle. Outside of advanced LWRs, which use Low-Enriched Uranium (“LEU”) fuel identical to or slightly modified from today’s existing reactor fleet (the use of existing fuel designs being one of their largest selling points, in addition to leveraging a further defined regulatory framework), many of the most promising new advanced nuclear technologies will depend on High Assay, Low-Enriched Uranium (“HALEU”) for fuel.
HALEU is Uranium where the amount of fissile isotope (Uranium-235) is enriched to above the 5% threshold of LEU and below the 20% threshold of High-Enriched Uranium (“HEU”)[iii]. In theory, this could have just been called “Medium-Enriched Uranium” but that would have gone against the clearly defined industry practice of opting for acronym complexity wherever possible to retain an aura of inaccessibility to the general public (note: humor). Some benefits of using HALEU with new fuel designs include the ability to achieve increased power density, longer core lives, improved economics, less waste, and enhanced safety. While HALEU holds tremendous promise for the industry, the fuel cycle supporting it remains in its infancy. Today, the only supplier capable of delivering HALEU in the near term is a subsidiary of the Russian nuclear national champion, Rosatom. While many reactor developers had planned to source initial cores from Rosatom, current geopolitical dynamics make this impossible. The result: Today the #1 issue on the minds of most advanced reactor developers and customers is on fuel sourcing and availability. Let’s dig in…
It is important to realize that the current situation is more a result of commercial incentives than technological limitations. Countries in North America and Europe have the technology to build facilities which produce HALEU. However, given that customer orderbooks for advanced reactors are still being built out, the current market lacks enough commercial visibility on demand to compensate would-be producers for the risk associated with long lived investments that have significant up-front capital costs. This is one of the many chicken-and-egg problems the nuclear industry faces today – reactor customers want to see fuel security to commit to a particular reactor design, but fuel cycle investments are dependent on the customer base placing reactor orders to firm up demand projections. The solution currently under consideration is for governments to help de-risk those investments by acting as a buyer of HALEU in the initial years of the industry’s development (the government can then sell that HALEU back into the market as demand is confirmed). This is a plan that Segra wholeheartedly supports and a great example of where a small investment by government can have a lasting impact on essential clean energy infrastructure. In fact, the U.S. Department of Energy will be issuing a formal request for proposals for HALEU production before year end, which should push at least two potential producers toward commercialization (Urenco, Centrus, and Global Laser Enrichment / Silex will be vying for this opportunity).
The development of HALEU and deployment of advanced nuclear technology will have far reaching implications for the existing nuclear fuel cycle, and while many investors and market commentators still view that impact as in the distant future, we believe it will begin to impact utility procurement in the relatively near term. As we have stated in past musings, understanding the timelines associated with the nuclear industry is essential to forecasting the market effectively. A common mistake among forecasters is to focus on near term supply / demand balances and assume they will drive market dynamics.
A common bear case for uranium is that in the very near term (2022 – 2023) utilities have relatively low uncovered requirements, and that true sizable deficits only begin to appear in forecasting models after 2025. Our response is that near term coverage has always been high and that has never stopped a price cycle from occurring. Utilities are relatively well covered in 2022 and 2023 because they bought that material 3 – 5 years ahead of needing it (and they always will). Without re-hashing the basics on utility fuel contracting, it is important to emphasize that new uranium mines often take 10 years or more from discovery to production, and once uranium is mined it needs to be transported, converted, enriched, fabricated, and delivered to a customer before it can enter a reactor (a process that start to finish often takes up to two years). Building out capacity for conversion and enrichment have similar large capital requirements and long timelines. Don’t take our word for it – below is a slide from the largest nuclear operator in the United States, Constellation Energy. It shows that they begin contracting for reload requirements 6 years prior to refueling dates and target full coverage at least two years before a refueling.
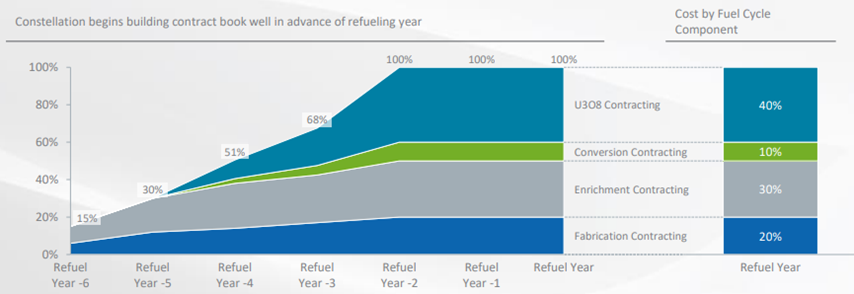
While every utility customer is different, in our work we have seen that procurement supporting a new reactor or first core often takes place several years before start-up to minimize market risk (new reactors in the UAE were a great example of this). Going back to nuclear 101 – once a reactor is up and running, a sure way to destroy plant economics is to face unforced outages due to fuel availability issues. This is a worst-case scenario for operators and also why security of supply becomes such an extreme focus during times of market stress (this will be as true for advanced nuclear as it is for traditional LWRs).
What’s the point of all this? While many market participants continue to see the demand profile of advanced nuclear as some distant factor, we see it’s incorporation into long-term forecasts as a key variable which will drastically shift models today. Let’s talk about why.
First, many forecasting models for advanced nuclear show initial units being built in the 2020s with a relatively sharp ramp up in deployment throughout the 2030s. Again, because uranium is typically mined at least 2-3 years before it is inserted into a reactor core, that ramp will impact supply and demand in the back half of this decade (a period which utilities are beginning to contract for now, and during which most forecasters are projecting significant deficits across fuel cycle components). The procurement of HALEU will clearly impact the uranium and conversion markets, but it may also drastically impact the enrichment market for LEU. While new HALEU production facilities will be built in the relatively near future, those projects will likely begin with LEU rather than un-enriched uranium feedstock (there are obvious benefits of enriching fuel from 5% to ~20% rather than starting with natural uranium). This means the entire fuel cycle will tighten just at the point where supply and demand look most strained today.
Second, and perhaps most importantly, new reactor and fuel designs mean that the procurement cadence for advanced reactors could look quite different from the world fuel buyers operate in today. One of the major selling points of many advanced reactors is their ability to operate with significantly elongated refueling cycles. We have reviewed dozens of reactor designs but today we will highlight just a select few to outline this dynamic.
X-Energy’s Xe-100 is a High Temperature Gas Cooled Reactor operating on a thermal spectrum with an outlet temperature of ~750 degrees Celsius. The high heat steam produced by this design makes it uniquely suited to commercial deployment supporting industrial processes and hydrogen production. The design is an 80 MWe pebble-bed reactor that uses inert helium as the coolant and energy transfer mechanism. Units can be packaged to meet customer demands with initial buildouts forecasted to be 4-packs. Fuel is a HALEU based TRISO design and refueling will occur online while the reactor is operating. TRISO fuels are structurally more resistant to neutron irradiation, corrosion, oxidation and high temperatures (the factors that most impact fuel performance) than traditional reactor fuels. Fuel production is envisioned to take place at centralized locations and X-Energy’s TRISO fuel can be utilized by a number of different Advanced Reactors designs. Fuel cycle implications are hard to predict here given the centralized nature of fuel production, but the online refueling suggests a smoother demand profile than other advanced designs. X-Energy was also awarded $1.25 Billion under the U.S. Department of Energy’s (“DOE’s”) Advanced Reactor Demonstration Program (“ARDP”), and they are building their initial reactor with utility partners Energy Northwest and Grant County PUD in Washington State (Operation expected in 2027).
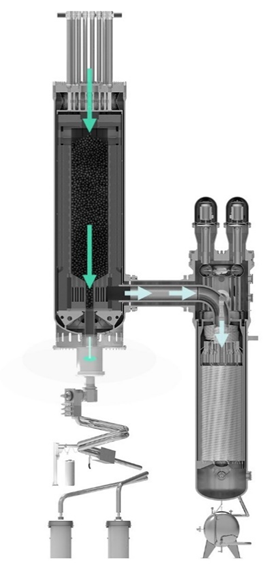
Bill Gates’ TerraPower teamed up with GE-Hitachi to develop the Natrium Reactor. The design is a ~345 MWe fast reactor that utilizes liquid sodium as a heat transfer mechanism paired with a large molten salt heat sink which operates as a grid scale battery allowing the plant to vary output from ~100 MWe to ~500 MWe. Reactor fuel is a HALEU based metallic Uranium-Zirconium design with significantly longer in reactor lifetimes than traditional reactor fuel. One benefit of the fuel design is that approximately 1/7th of the core is replaced each 24-month refueling cycle which drives higher burnups than traditional nuclear reactors and a more efficient fuel cycle. This higher burnup means that Natrium reactors produce 2/3 less waste than traditional light-water reactors. The fuel cycle implications of this design will be for a much larger first core procurement and smaller per-refueling reload requirements than traditional light-water reactors. Natrium was awarded $1.25 billion in funding from the U.S. DOE’s ARDP and are building their first reactor in Kemmerer Wyoming with utility partner Pacificorp (Operation expected in 2028).
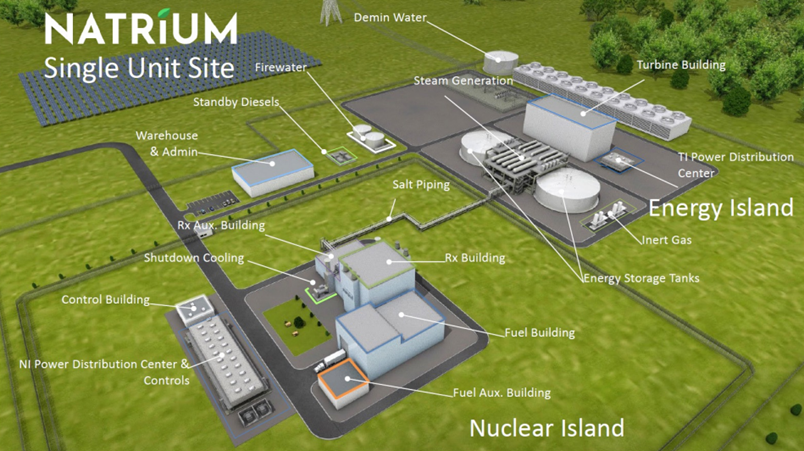
ARC Clean Energy’s ARC-100 is another exciting fast reactor also utilizing liquid sodium for heat transfer. The 100MWe design borrows many of the concepts initially developed by the Integral Fast Reactor program developed in the U.S. national labs programs beginning in the 1960s. Like Natrium, it uses a HALEU based metallic Uranium-Zirconium fuel design but is expected to run for up to 20 years without refueling. The benefits of a prolonged refueling cycle are significant both for fuel efficiency, and for deployment cases in emerging market countries that lack the infrastructure or expertise to coordinate more frequent 18-24 month cycles. The fuel cycle implications of this design are clearly significant as the reactor’s first core would require 20 years of reactor fuel, making the demand profile far more front-loaded than traditional LWRs. ARC has won funding from the U.S. DOE under the ARDP in the ARC-20 funding line as well as from the Province of New Brunswick, Canada. It is building its first reactor at the Point Lepreau Project with utility partner NB Power (Operation expected 2029).
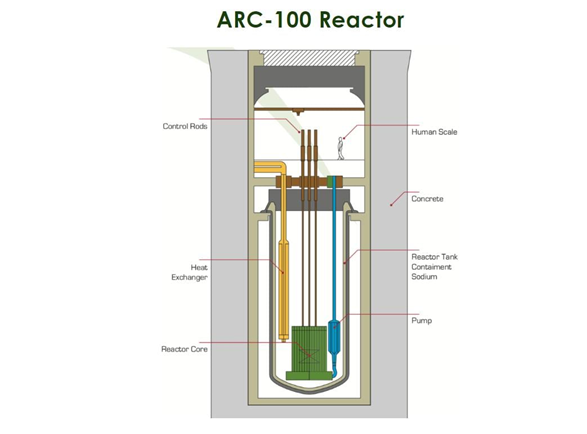
Hopefully the brief summaries above give you some flavor for the variety of reactor designs and fuels currently in development today (we count upwards of 70 different reactor designs and close to 150 private advanced nuclear companies today, and the opportunity set keeps growing – this is just the tip of the iceberg!). The implications for the fuel cycle should be apparent. For example, while a 100MWe advanced reactor may not seem like a big demand driver, if you are deploying them in 2 or 4 packs and they require 10 or 20 years of fuel up front, the impact on uranium markets can be quite substantial (while we cannot disclose firm first core estimates for the designs we have reviewed, we’re often talking multi-million-pound initial cores). Given the front-loaded nature of fuel requirements, the roll out of these designs in the 2030s will have significant fuel cycle implications.
Following first of a kind reactor deployment, each of these companies are targeting dozens of deployments from the 2030’s onward. The growing demand slice and lumpiness of procurement is something that will undoubtedly shift market forecasts substantially as orderbooks build. The next logical question is – how are forecasters modelling this today? The answer – not in any concrete way.
First, the World Nuclear Association’s 2021 World Nuclear Fuel Report (of which we are a proud contributor) largely tabled the advanced nuclear discussion until the industry has access to better data around reactor deployments and fuel designs. We understand this given that some of this information is not publicly disclosed and inserting a theoretical reactor design as a placeholder introduces all sorts of issues as the model is further refined over time. Our conversations with industry consultants thus far have led us down different pathways. Initial discussions with one well-known consultant led to a very open discussion around (1) how incredibly important this piece of the puzzle is to effectively forecast the fuel cycle, (2) an acknowledgement that they are still developing their framework on this topic, and (3) an offer to share views and modelling techniques with each other in the future. Our email exchanges with the other industry consultants involved broader disregard and the usual smoke, mirrors, and assurances that “it’s in the model” (an assertion we remain skeptical of given the smoothness of their demand profile lines in what will undoubtedly be very lumpy procurement timeframes).
One targeted study on HALEU requirements – Estimated HALEU Requirements for Advanced Reactors to Support a NetZero Emissions Economy by 2050 – was prepared by Idaho National Lab’s Integrated Energy and Market Analysis team for the U.S. DOE. The report forecasts different HALEU demand profiles based on a series of modeling constraints driving a Net-Zero by 2050 target. We would note that the analysis was performed in 2021, prior to Russia’s invasion of Ukraine, which has accelerated interest in advanced nuclear dramatically. Additionally, the deployable Advanced Reactor technologies in their model were constrained to two design types (X-Energy’s Xe-100 and TerraPower’s Natrium) and the study assumed that new nuclear construction would be roughly equally split between traditional GW-scale Light Water Reactors (AP-1000 design), NuScale Power’s advanced LWR design, X-Energy’s Xe-100, and Terrapower’s Natrium. We would argue that (1) the study’s limitation to two advanced reactor designs oversimplifies a quickly changing market and (2) the assumption that we are going to build a significant number of new GW-scale LWRs despite advanced nuclear technology being commercialized may be unrealistic given (much to our chagrin) the customer base’s current aversion to infrastructure projects of that scale. That being said – it’s easy to nit-pick someone else’s model and forecasting on this scale is difficult so let’s just look at the results:
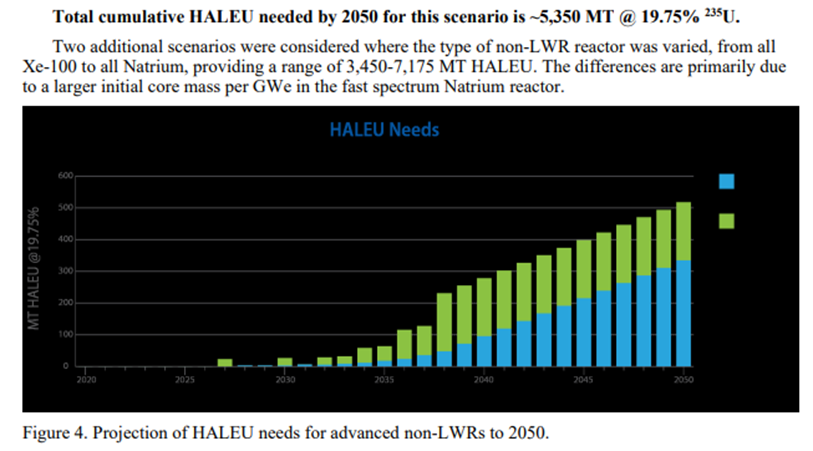
The model projects demand for HALEU increasing to over 500MT per year under its mid-case (and significantly more if deployment is more heavily weighted to the Natrium reactor with a larger initial core mass requirement). This would effectively double the United State’s annual uranium and conversion demand by 2050. The impact on enrichment is slightly harder to forecast due to the cascading effect of HALEU demand for LEU potentially shifting optimal tails for the entire enrichment market (but this is a rabbit hole we’ll save for another day). Let’s also be clear that this only covers the U.S. market and likely understates HALEU demand by assuming that 50% of next generation nuclear deployments will be based on LWR designs (this point is debatable, but we’d bet against it).
This all paints a picture where we see significant growth in fuel cycle demand (exacerbated by the front-loaded nature of new technologies) in the late 2020s and early 2030s right at a time where uranium, conversion and enrichment are already facing a supply/demand imbalance from the current global reactor fleet.
Concluding Thoughts and Takeaways
Segra Capital has been focused on the nuclear markets in one way or another for close to a decade, and completely dedicated since 2018. We can honestly say we have never been more excited about the prospects for nuclear power. To borrow an overused line, nuclear’s future is brighter than ever!
With that said, we fear that shortsightedness, oversimplified forecasting, and perverse market incentives could unnecessarily hurt the industry’s ability to deliver on its promise to society. In Segra’s view, 2030 might as well be tomorrow for the nuclear industry. Governments and industry participants need to understand the competitive landscape unfolding in today’s marketplace (China and Russia are building their own advanced reactors, both for domestic use and export and whether we like it or not, they’re in the lead!) and be ready to send the commercial signals which incentivize investment across the nuclear fuel cycle. We strongly encourage the U.S. Government to appropriate the necessary funds to support a healthy and secure domestic nuclear fuel cycle (for today’s fleet and tomorrow’s) beginning with HALEU production. We would also prod market participants to realize the risk they are taking if they don’t incentivize great projects with great partners today.
We will continue to update the market with our views across the nuclear landscape in the future. We would encourage everyone to get some rest with their families this summer if they can. While we don’t have a crystal ball, everything we’re seeing suggests the industry is in for an exciting and eventful fall.
Thanks for reading,
Segra Capital Management
[i] We want to be clear that we are BIG fans of traditional, large scale LWR nuclear power plants (and we hope the world builds many more of them). After spending years focused on nuclear, we’ve come to believe that one of the main challenges for the industry is the tribal, siloed nature of some of its strongest advocates. We see this repeatedly – groups of experts who only believe in one select technology (Large vs. Small, water vs. liquid metal or molten salt, uranium vs. thorium… etc). We believe this has real implications for the sharing of best practices and for the industry’s ability to form a unified message about the technology’s promise to potential stakeholders. At Segra, our ability to look across technologies is an edge that we believe both our investors and portfolio companies will benefit from over time.
[ii] While a portion of our venture fund will look at opportunities in nuclear fusion, the majority of this blog post will focus on advanced fission. To hear more about our fusion views, click here.
[iii] Sometimes the industry refers to enrichment between 5% and 10% as “LEU+” rather than HALEU